15 February 2016
Sumner rockfalls – videos from the M=5.7 earthquake near Christchurch yesterday
Sumner rockfalls
The M=5.7 earthquake in Christchurch, New Zealand yesterday triggered a new set of rockfalls in the Sumner area to the south of the city. These Sumner rockfalls occurred on the same cliffs that were subjected to repeated collapses in the 2011-12 Christchurch earthquake sequence. I have posted images of these rockfalls previously, and there has always been concern that there would be further collapses on the cliffs to the south of the city as a result of further aftershocks or intense rainfall.
The earthquake is well-described in a press release put out by Geonet:
A magnitude 5.7 earthquake has occurred at 1:13 pm New Zealand time, 15 km east of Christchurch approximately 15 km deep. So far we have received 2974 felt reports from Hawke’s Bay to Invercargill as at 6:30 pm. The highest level of impact from our felt reports so far has been MMI 8 in central and Christchurch. This is also shown in the coloured Shake Map … which models shaking intensity in Modified Mercalli Intensity.
This is the Shake Map that Geonet have posted. Expect this to be updated in the days ahead, but it shows that parts of the Christchurch area were subjected to quite intense shaking:
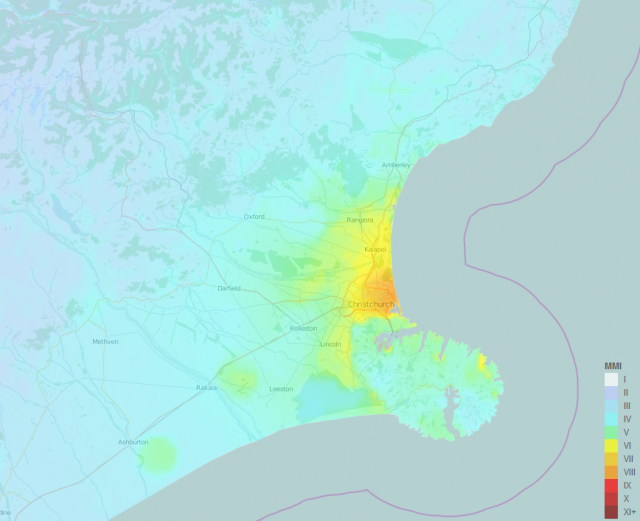
Geonet shakemap from the M=5.7 earthquake off the cost of Christchurch on Sunday
.
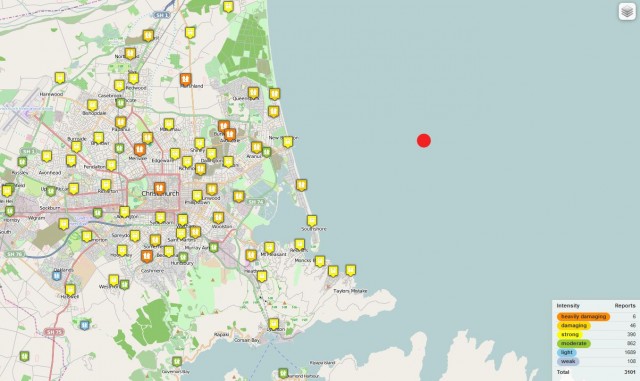
Geonet map showing felt reports in the Christchurch area
.
As usual the felt reports correlate well with the shaking intensity map. The Sumner area was subjected to strong shaking, triggering the Sumner rockfalls. The timing of the earthquake was perfect for the collection of videos – it occurred on a Sunday afternoon in the summer vacation on a sunny day near to the beach. In consequence several people captured videos and photos of the Sumner rockfalls. The best photo is this one I think, taken by RNZ / Sally Murphy, shows the rockfalls in action. Note that the images shows multiple streams of shallow rockslides and rockfalls, all generating large amounts of dust:
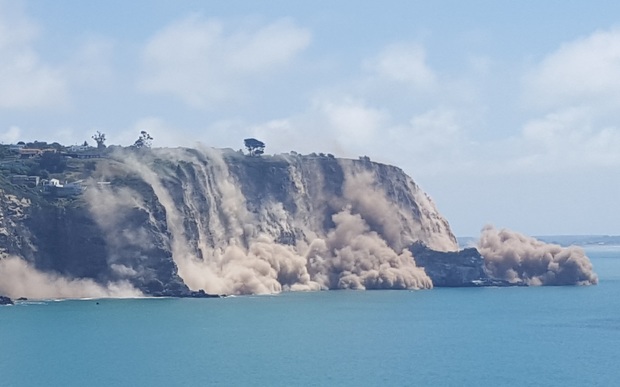
Sumner rockfalls by RNZ/ Sally Murphy
.
There is a host of videos on Youtube showing the immediate aftermath of the rockfalls. Three of the best examples are as follows:
.
.
.
Amazingly there is also drone footage available already showing the sites of these rockfalls:
.
The volume of material released in these Sumner rockfalls is not huge, but there are many sites on the cliffs. In the next few months there will be a new burst of aftershocks, so expect more rockfalls, albeit on a lesser scale.
10 February 2016
Fude Village, China: six killed in a rockslide
Fude Village rockslide
Early on Monday morning a rockslide occurred at Fude Village in Du’an Yao Autonomous County in Guangxi Zhuang Autonomous Region, in the south of China. The Chinese news agency Xinhua has a report and images off the landslide, which it describes as follows:
The emergency response office in Du’an Yao Autonomous County under Hechi city received information at 3:25 a.m. Monday that a two-story building in Fude village collapsed in a landslide. Lan Min [a] villager, was sleeping when the accident happened. He was woke up by a loud noise, which he mistook as fireworks at first. “But then I felt the house was shaking,” he recalled. “I thought it was an earthquake and told my family to rush out.” When they stopped, they looked back, seeing Lan Huajing’s house entirely destroyed, and a wall of their own house damaged by stones.
This Xinhua image shows both the source of the landslide and the track, together with the house that the debris struck:
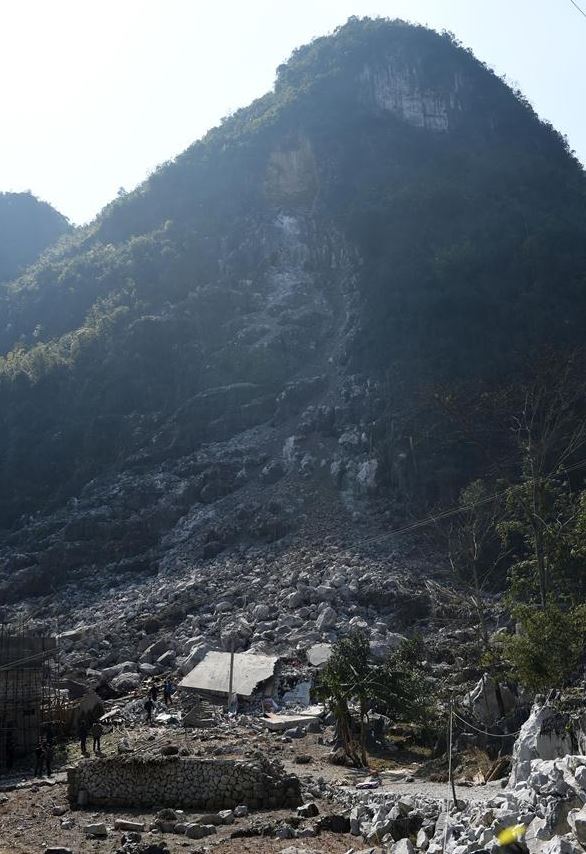
The rockslide that killed six people in Fude village, Guangxi Zhuang Autonomous Region, China on Monday 8th February 2016. Image via Xinhua
.
There are a couple of things that are interesting here. First, the location of the original rockfall is clear. The volume of the debris at the toe of the slope looks to be substantial (although some may originate from earlier rockfall events), suggesting that the landslide entrained other debris en route down the slope. Second, note that the scar from the source of the rockfall is heavily discoloured compared with the freshly fractured rock downslope. This suggests that the rockfall originated from a pre-existing joint or fracture. The news reports suggest that this was not triggered by rainfall.
The deaths occurred in a single property that was impacted by the debris. The damage to the house is dreadful:
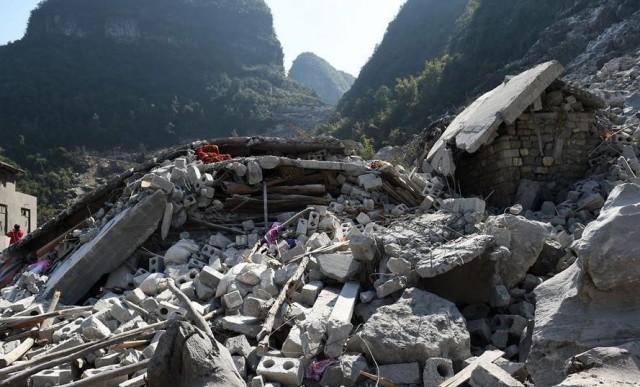
The house destroyed by the rockslide at Fude village Guangxi Zhuang Autonomous Region, China. Image via Xinhua
8 February 2016
Taking responsibility for disaster risk reduction
Taking responsibility for disaster prevention
Disaster prevention, regardless of the peril, works through many small actions by individuals, every one of whom must take individual responsibility. To achieve a genuine increase in resilience requires dedication, coordination and determination, based on sound judgement, good science and (in many cases) high quality engineering. Sadly, the flip side of this is that individuals can undermine the efforts of everyone else, and in so doing turn a survivable incident into a disaster.
The case of the Taiwan earthquake appears to be a good example. The collapse of the Weiguan Jinlong apartment complex should not have occurred – a building of this type should have been able to withstand the peak ground accelerations associated with an earthquake of this magnitude, even allowing for the potential for some amplification from the sediments under the foundation. The collapse is quite odd in many ways – the building has clearly toppled over. But over the last day or so images have emerged of the state of the key columns that supported the building, and the images are shocking:
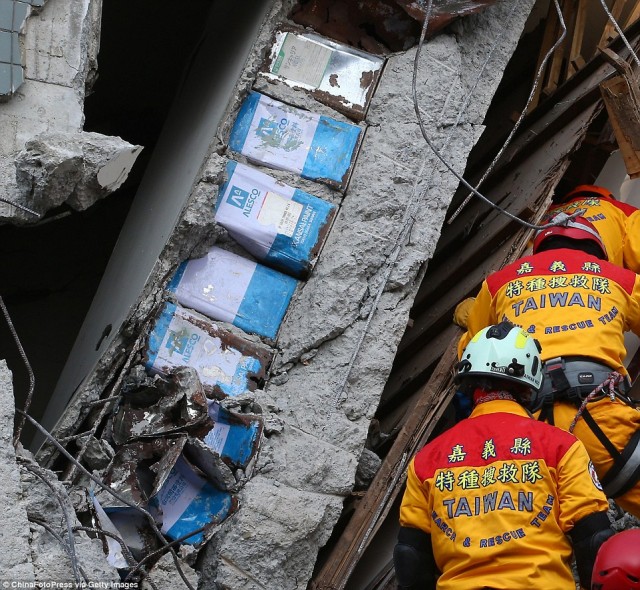
Tin cans within the structural columns in the Weiguan Jinlong apartment complex in Taiwan (via China Foto Press)
.
The apparent replacement of structural concrete with empty cooking oil cans may have critically weakened the building, and if these are load-bearing may have played a role in the failure. The cans provide almost no structural strength, and of course mean that the amount of rebar within the concrete is also reduced. Interestingly, there is surprisingly little rebar in the section of the column that does have concrete.
And this column is not alone. This Taipei Times image shows a double layer of cooling oil cans:
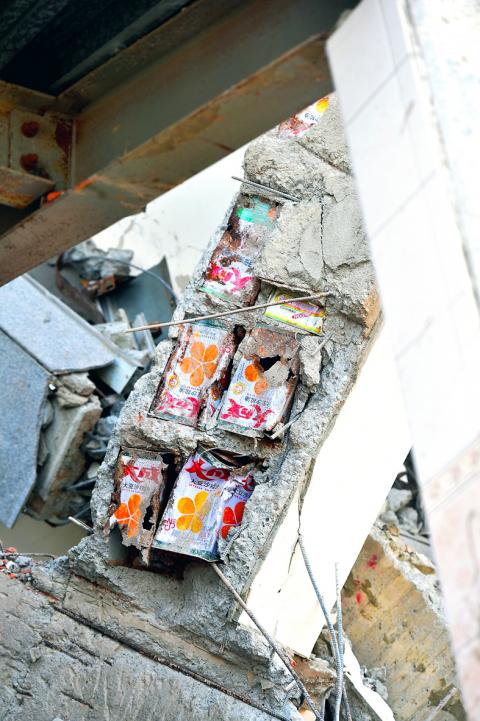
Cooking oil cans in columns in the Weiguan Jinlong apartment building collapse, via the Taipei Times
.
And of course if this was going on it is likely that there were other problems with the building too, and these problems may have been the real cause of the collapse. The irony is that the saving here was not huge in the context of the cost of the building – some rebar work, some cubic metres of concrete. But this is not the action of one person – to place cooking oil cans in the columns like this would have required the collusion of several individuals at least.
Meanwhile, in Brazil the investigation into the Samarco tailings dam disaster that devastated Bento Rodrigues late last year continues. This will be watched with keen interest – the mining industry has a wretched track record with tailings dam failures – I cannot think of any other engineered structure that has such a high failure rate, especially in light of the devastating consequences. The Brazilian government is now estimating that the impact of the failure, excluding the environmental costs, are now over $300 million. And for once the authorities are responding by taking a very hard line. Telesur reported as follows last week:
Brazilian authorities said they have gathered enough evidence to charge Brazilian mining executives with murder after 19 died in the tragedy. Brazilian police authorities announced Friday that they have gathered “sufficient evidence” in order to charge high-ranking executives from Brazilian mining companies with murder, following the aftermath of the November 2015 dam burst in Minas Gerais state. “We have gathered all of the findings from the autopsy reports, in which we determined that the crime of murder had been committed,” said Rodrigo Bustamante, who has been heading up the investigation for the state’s civil police.
A charge of murder will raise eyebrows for sure. I cannot comment on the likely outcome of the case, but such strong legal action should force mining executives to start to take proper responsibility for their tailings dams. That must be a good thing.
If we are to reduce losses from natural hazards, whether they are earthquake, landslides or floods, then there is a need for individuals to take responsibility.
4 February 2016
Landslides near Muzaffarabad from the 2005 Kashmir earthquake
Landslides near Muzaffarabad from the 2005 Kashmir earthquake
The M=7.6 2005 Kashmir earthquake in northern Pakistan is thought to have killed over 80,000 people (and most likely over 100,000), and left 3.5 million people homeless. A key factor in the impact of this earthquake was the devastating effect of many thousands of landslides. One of the most seriously affected areas was Muzaffarabad – the city itself was very seriously damaged by the shaking, and there was also a huge impact of landslides in the local area. Google Earth now has very high quality imagery of the area before and after the earthquake, and a further set collected more recently (in 2014), almost a decade after the event. This is an image of one of the most seriously affected areas before the earthquake, taken in 2002:
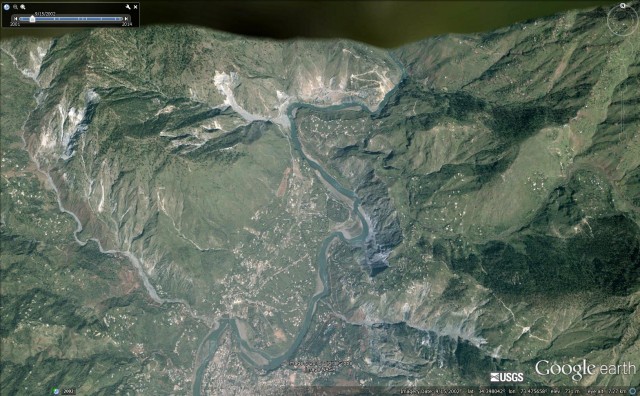
Google Earth imagery of the Muzaffarabad area in 2002, before the 2005 earthquake
.
The river that runs north – south across the image is the Neelum. On the southern edge of the image the main part of the city of Muzaffarabad is clearly visible. There are landslides in the image, most notably along the Neelum and on the walls of some of the smaller channels. Contrast this with an image of the area after the October 2005 earthquake – this image was collected on 31st June 2006. Note that the green area on the right is the pre-earthquake image:-
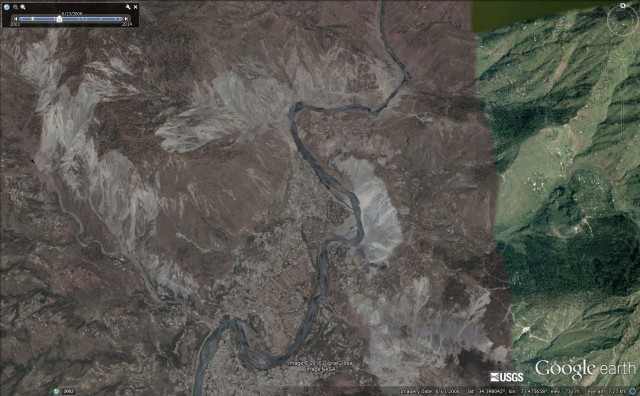
Google Earth imagery from 2006 showing the dramatic increase in the amount of landslides triggered by the 2005 earthquake
.
The most dramatic area of landsliding is the huge cliff collapse events that occurred on the east bank of the river to the north of the city (and note how much the city had expended in the few years prior to the earthquake), but there is also dramatic landsliding along the Neelum river, to the east of Muzaffarabad and along the river to the northwest. I took photographs of some of the landslides at the time. This is the area of massive rock cliff collapse to the east of the city:-
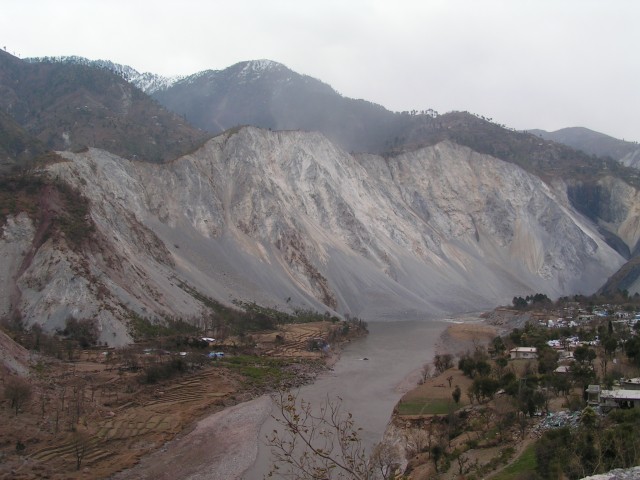
Massive rockslope failure to the east of Muzaffarabad
.
Whilst this some of the landslides on the Neelum River. This area was extremely badly affected my shallow rockslope failures:
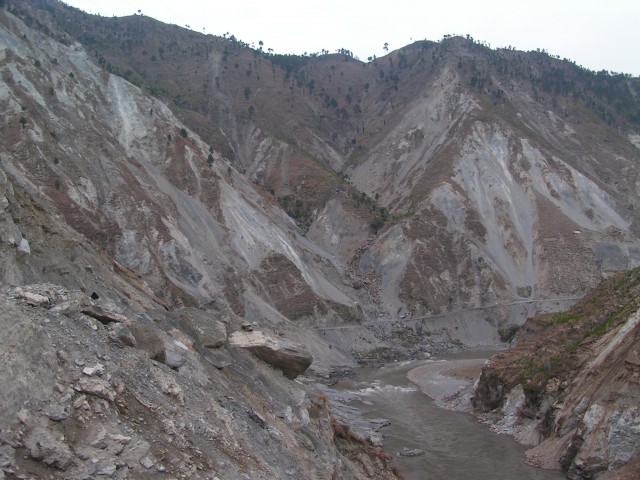
Extensive shallow rockslides along the Neelum River
.
Landslides are one of the enduring legacies of an earthquake in a mountain chain. This Google Earth image was collected in April 2014. It is heartening to see reconstruction in the city, but note just how obvious the landslides are, and many are very obviously fresh failures. A decade on it is clear that earthquake induced landslide remain a major issue in the Muzaffarabad area:
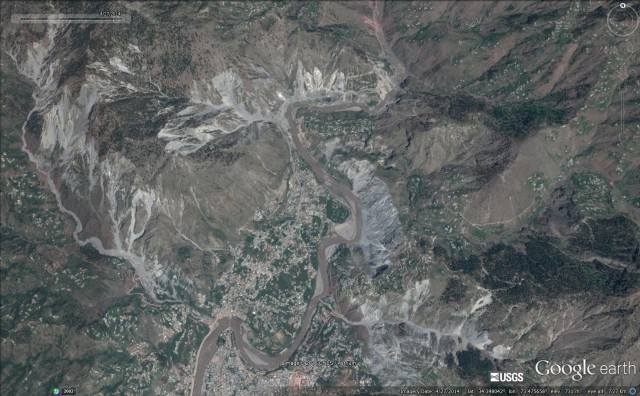
Google Earth imagery of the Muzaffarabad area, collected in 2014
3 February 2016
New landslide videos: Aconcagua (again), Muothatal and Pacifica
Another debris flow on Aconcagua
Last week I blogged about a debris flow video on Aconcagua in Argentina. It turns out there is another video of an earlier debris flow, collected on 26th December 2015:
The text associated with the video, which was shot by Kyle Peterson, is as follows:
I shot this video Dec 26, 2015 and our group nearly got caught in a rock/mudslide hiking out of Horcones Valley after summitting Aconcagua the previous day.
This video beautifully illustrates that dry nature of these types of flow, even though they look wet. Look at the amount of dust the flow is generating as the dry rocks scrape along the walls of the channel:
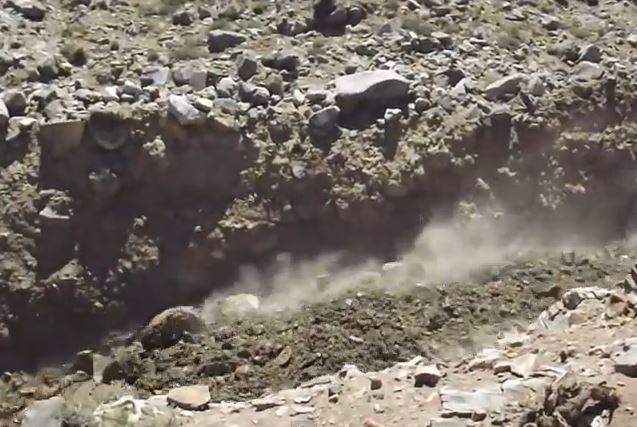
The 26th December 2015 debris flow on Aconcagua in Argentina. Still from a Youtube video collected by Kyle Peterson
.
I’d be very interested to know the source of these debris flows.
A fascinating landslide at Muothatal in Switzerland
Meanwhile, this amazing landslide was apparently triggered by a combination of rain and snow at Muothatal in Switzerland:
.
Drone footage of coastal erosion at Pacifica
Repeated El Nino storms have driven more rapid erosion on the bluffs at Pacifica in central North California. The resultant erosion appears to be condemning a number of buildings, as very impressive drone footage shows:
2 February 2016
Landslides in Chile Part 5: Water waves triggered by landslides and the Mentirosa Island Landslide complex
Landslide in Chile 5: the Mentirosa Island landslide complex
By Dr Melanie Froude (@Froudo_baggins), University of East Anglia
Cymophobia, the fear of waves and wave-like motions, is capitalised on by the 2015 Norwegian film, The Wave (originally titled Bølgen). Opening sequences of the trailer remind us of the devastating Norwegian Tafjord slide of 1934. Here an estimated 3 million m3 of rock slid into the fjord, generating a <60 m displacement wave of water that travelled 4.5 km up-fjord; inundating shoreline communities with c.15 vertical metres of water (Baathen et al., 2004). Distinction is made between the term displacement wave and tsunami, the former generated by subaerial landslides impacting into water bodies, and the latter caused by the movement of submarine landslides (Hermanns et al., 2014). With all the merits of a blockbuster disaster movie, The Wave presents a worst case scenario, fully CGI-realised, depiction of the catastrophic failure of the Åknes rockslide (also in Norway) and subsequent displacement wave. It is no surprise that this landslide has gained attention from filmmakers, having a colossal estimated volume of 30-40 million m3, and the potential to deliver a 40 m wall of water to the villages of Hellesylt and Geiranger (Oppikofer et al., 2009). The Åknes rockslide is one of the most extensively monitored landslides in the world, and alarm thresholds with public alert levels have been established based on displacement, velocity and acceleration of the slope surface and subsurface (via boreholes).
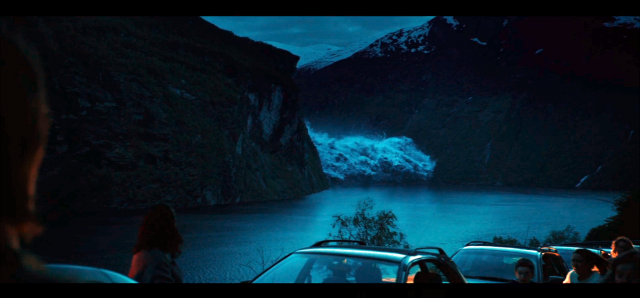
Frame from The Wave. Tsunami rounds the bend of the fjord generating panic for evacuating residents of Geiranger. Film due for US full-release on 4th March 2016, IMDB
.
Water waves triggered by landslides, either originating from an exposed slope (e.g. valley wall) or occurring under water (submarine), pose a danger to communities living around confined water bodies such as fjords, coastal bays, rivers, lakes and reservoirs. Large masses of rock are effective at generating waves when impacting water at high speeds. Waves are then guided by local narrow topography, which may amplify wave height and reflect waves sending them in an alternative direction; leading wave activity to last for hours and extensive damage to shorelines from run-up. Several landslides that triggered waves of different magnitudes have been reported in this blog: (1) Porcupine Bay, Washington State, (2) Nord-Statland, Norway, (3) Vaiont, Italy, (4) Daning River, China, (5) Sokjosen, Norway and (6) Lituya Bay, Alaska. The largest wave occurred in Lituya Bay and had a maximum run-up height (vertically up the valley wall) of 530 metres. Sonny and Howard Ulrich provide an eyewitness account of the event for the BBC Mega Tsunami documentary, available here.
Differences in source landslide trigger, size, material and mechanism, as well as local variations in topography, challenge scientists who use numerical computer models to forecast the formation and propagation of water waves to inform hazard assessment. There is much research published in this field for sites in the fjords of Norway, which is unsurprising as Oppikofer et al. (2009) point out, historical and geological evidence indicates one catastrophic rockslide event has occurred approximately every 100 years in the Storfjord in Western Norway alone. Shifting hemispheres, and focusing on the fjords of Chilean Patagonia, I was surprised that water waves triggered by landslides were not more commonly reported given the extensive network of fjords containing steep slopes of often fractured bedrock and volcanic surficial deposits. Landslides may be occurring out of sight of coastal population centres, historic events may not be documented due to the relatively recent mass settlement of the region (following the 1902 Cordillera of the Andes Boundary Case), or the physical conditions for occurrence of a landslide of sufficient magnitude and mechanism to locally generate a displacement wave or tsunami, may not be often realised. With the exception of the landslides triggered by the Aysén (Aisén) earthquake on 21 April 2007, and a local testimony from Puerto Aysén regarding landslides and waves generated by an earthquake in November 1927 (Naranjo et al., 2009), very little is published on landslide-induced tsunamis/displacement waves in Chilean Patagonia. Of course, there is also the possibility that events and historical studies reported in Spanish have not been identified, and I welcome contributions from readers highlighting these.
The Mentirosa Island Landslide complex, Chilean Patagonia
My last blog post discussed the Punta Cola rock avalanche, providing some detail on Aysén (Aisén) fjord and the 2007 earthquake on the Liquiñe-Ofqui Fault zone (LOFZ). The Mentirosa Island landslide complex is composed of four soil-rock slides from the north-eastern slopes of Aysén fjord, with a total estimated volume of 8 million m3 (Sepúlveda & Serey, 2009). It is located less than 5 km from the earthquake epicentre. Research on this landslide is ongoing by Sergio Sepúlveda and others at the University of Chile; observations here are preliminary from fieldwork in January 2016.
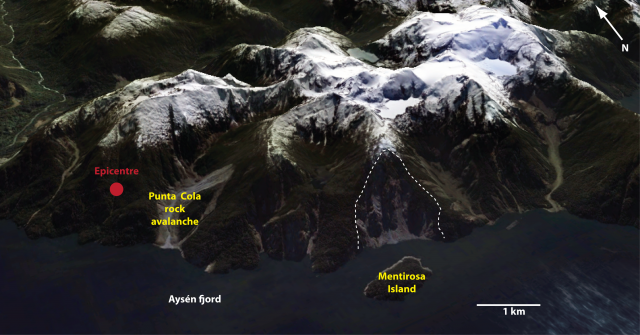
Location of the Mentirosa Island landslide complex, to the north of Mentirosa Island (shown within the dashed white line). The location of the 2007 Aysén earthquake epicentre is shown by the red dot.
.
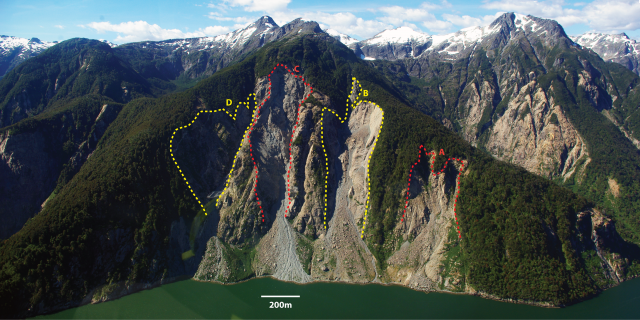
Four soil-rock slides make up the Mentirosa Island landslide complex. The source area for each is shown, and each scar is labelled (A to D). (Note there is a slight reflection in the photograph obscuring the lower section of D).
.
All four soil-rock slides failed from near the top of the hillside, suggesting the topographic amplification of seismic waves. Scar A appears to have derived from two smaller rock wedge failures, which have coalesced to form a debris fan at the slope base. Inspection of scar of B indicates the rock mass failed as a simple joint-controlled wedge, sliding downslope and disintegrating rapidly. The scar contains significant amounts of loose debris, stored on lower gradient rock ledges, showing the persistence of rockfall activity. Scar C has a slightly more complex geometry suggesting failure derived from two main wedges of rock; the translational movement is believed to be similar to B. The steeper geometry of the scar means debris is transported rapidly from rockslope to debris fan downslope. Large masses of highly fractured rock are exposed within the scar, highlighting the potential of future block failure. Landslide D is likely to have failed as a series of blocks; mineral veins are evident within the scar and the rock is highly fractured.
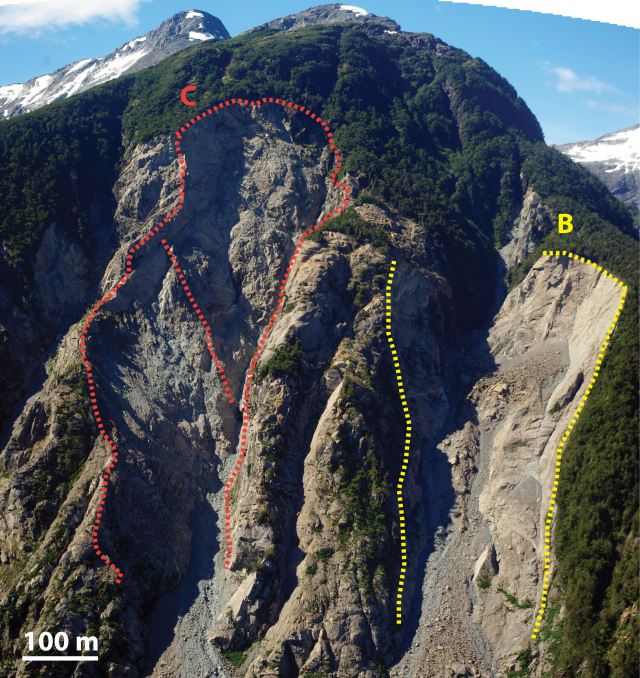
Mentirosa Island landslide scars B and C. Approximate boundaries of rock mass failure shown by dashed line. Red dashed line dividing the total scar area on C shows the possible division of the scar into two rock wedges.
.
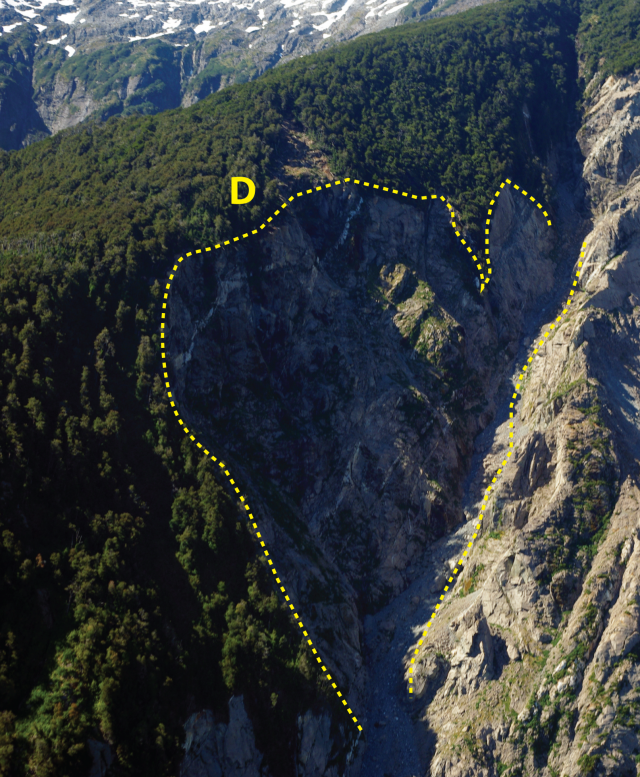
Mentirosa Island landslide scar D.
.
After detachment and disintegration it is possible that material from each soil-rock slide travelled downslope as a rock avalanche/ debris flow (depending on slope saturation) before impacting the fjord water surface. Fan deposits at the base of slide B show some inverse grading and larger boulders (~0.5 m) are located close to the surface. Material is predominantly angular gravels and boulders, and there is evidence for post-2007 rockfall and sediment-water flows (incision and cross-cutting channels) on the surface of the debris fan.
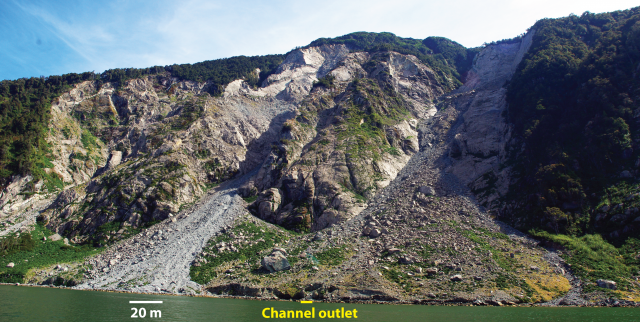
Looking up the debris fans of slide B (right) and slide C (left). Incision of channel into fan exposed vertical profile of landslide deposit (below).
.
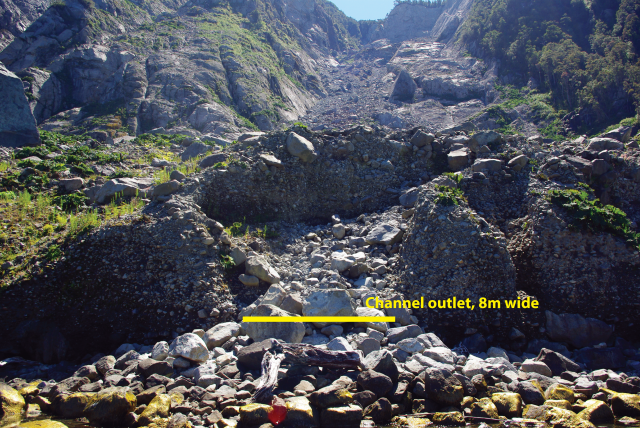
Looking up the debris fan of slide B
.
The impact of two of the landslides into the fjord were photographed by F. Olivera from a research vessel on the water at the time. These excellent photographs are published in Narangio et al.’s (2009) paper, which is free to view. Interpretation of the photographs suggests one of the landslides to the east of the Mentirosa Island complex impacted the water first (likely landslide B). The impact generated a displacement wave, which ran up the eastern coast of the Mentirosa Island. A second displacement wave generated by slide C impacted the island within 20 seconds of the first. The waves removed soil and vegetation from the lower <65 m of the eastern slopes of Mentirosa Island, and propagated into the fjord, increasing in amplitude in shallower waters, resulting in runouts of several hundreds of meters on coastal areas around Puerto Aysén. Landslides which triggered displacement waves and tsunamis were within 5 km of the epicentre of the 2007 Aysén earthquake. Seismic activity associated with the LOFZ poses a significant hazard to coastal communities in the Aysén region of Chilean Patagonia. The landslide and tsunami hazard for other towns such as Hornopirén, is being assessed using landslide inventories from the 2007 event and laboratory testing of slope materials, by members of the Newton Fund/NERC project on earthquake-induced landslides.
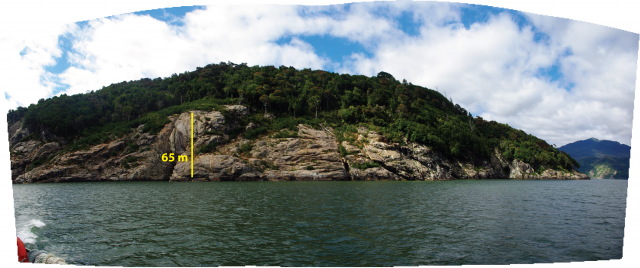
Mentirosa Island. Slope stripped of soil and vegetation from wave impact in 2007 still clearly delineated in 2016.
.
References
Baathan, A., Blikra, L.H., Berg, S.S. & Karlsen, F. (2004) Rock-slope failures in Norway; type, geometry, deformation mechanisms and stability, Norwegian Journal of Geology, 84 (1), pp.67-88
Hermanns, R.L., Oppikofer, T. Roberts, N.J. & Sandøy, G. (2014) Catalogue of Historical Displacement Waves and Landslide-Triggered Tsunamis in Norway (Chapter 13), in: Lollino, G., Manconi, A., Locat, J., Huang, Y., Artigas, M.C. (eds.) Engineering geology for Society and Territory- Volume 4: Marine and Coastal Processes, Springer, 235 pages
Naranjo, J.A., Arenas, M., Clavero, J., Muñoz, O. (2009) Mass movement-induced tsunamis: main effects during the Patagonian Fjordland seismic crisis in Aisén (45°25’S), Chile, Andean Geology, 36 (1), pp. 137-145
Oppikofer, T., Jaboyedoff, M., Blikra, L., Derron, M.-H., Metzger, R. (2009) Characterization and monitoring of the Åknes rockslide using terrestrial laser scanning, Natural Hazards and Earth System Sciences, 9, pp.1003-1019
Sepúlveda, S.A. and Serey, A. (2009) Tsunami-genic, earthquake-triggered rock slope failures during the April 21, 2007, Aisén earthquake, southern Chile (45.5° S). Andean Geology 36: 131-136. Doi: 10.4067/S0718-71062009000100010.
1 February 2016
Images of the Tbilisi Zoo landslide and flood disaster
Images of the Tbilisi Zoo landslide and flood disaster
Last week I posted Google Earth images of the Tbilisi Zoo landslide and flood disaster last year, and suggested that contrary to reports at the time, the losses were not caused by a valley blocking landslide, but rather a more conventional landslide that transitioned into a debris flow and combined with many other smaller events. Since then I have been contacted by Dr Sergey Chernomorets, who is Senior Scientist of the Laboratory of Snow Avalanches and Debris Flows in the Faculty of Geography at Lomonosov Moscow State University in Russia. He visited the site in September 2015 with Prof. Igor Bondyrev from Tbilisi State University and Elena Savernyuk, also from Lomonosov Moscow State University. Sergey confirms that the major cause of the disaster was the landslide at Akhaldaba village, and that there is no sign of valley blockage.
He also points out that the disaster at Tbilisi Zoo was a new road junction at Gmirta Moedani (Heroes Square), which dammed the flow.
Sergey has very kindly provided some photographs of the disaster, which I reproduce here with his permission. These two images show the landslide at Akhaldaba that initiated the Tbilisi zoo landslide and flood disaster:
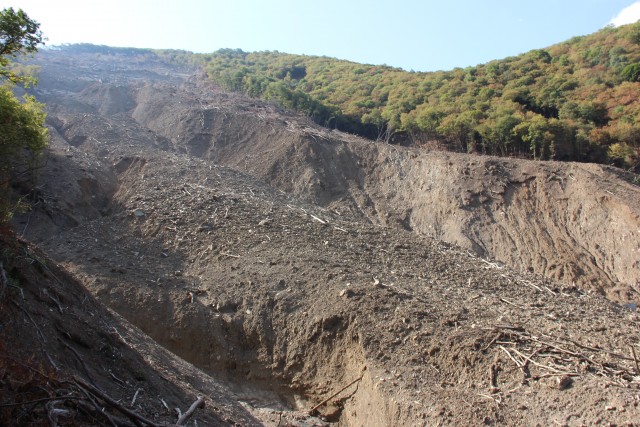
The Akhaldaba landslide courtesy of Dr Sergey Chernomorets
.
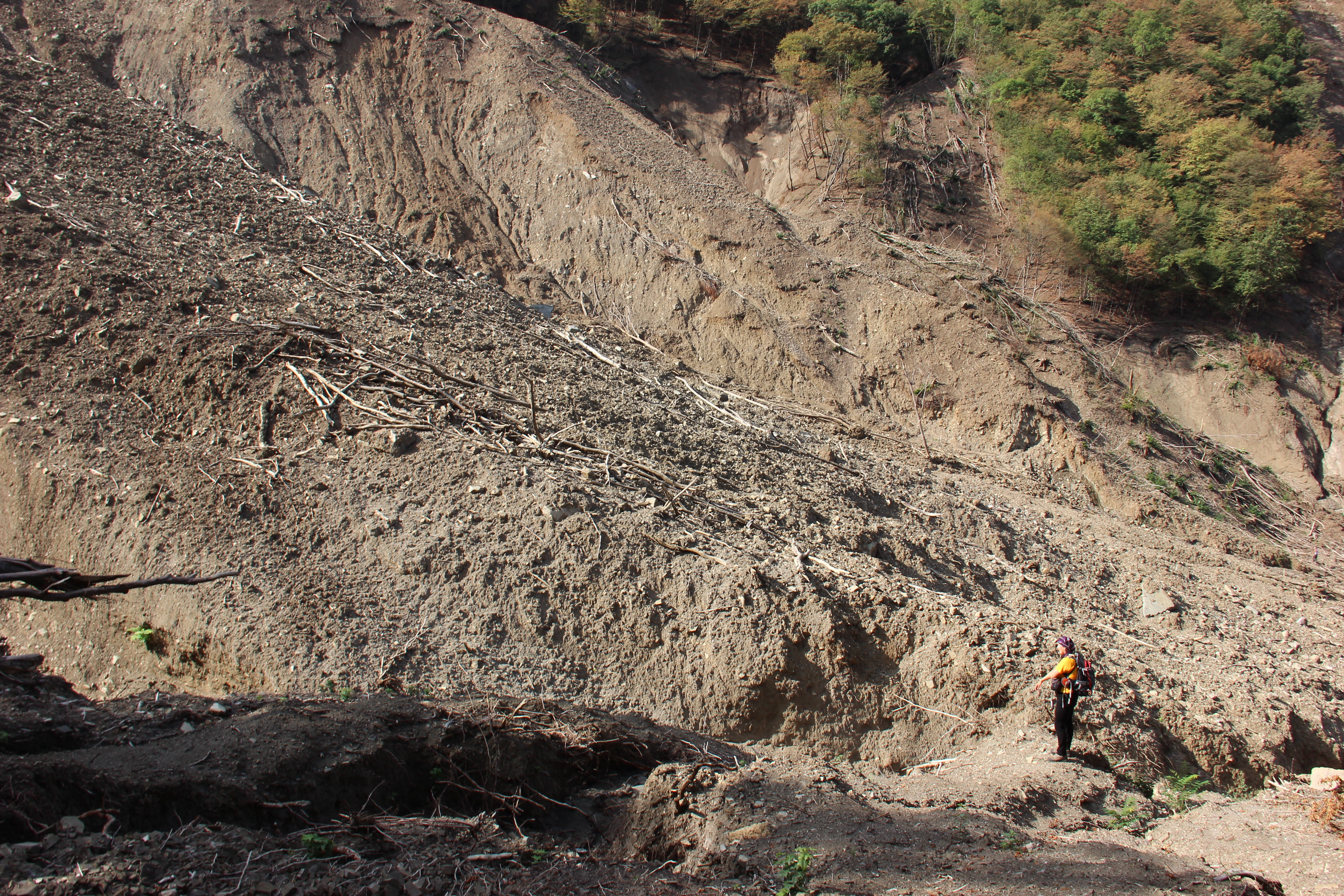
The Akhaldaba landslide courtesy of Dr Sergey Chernomorets
.
This is the road junction at Gmirta Moedani (Heroes Square) that dammed the flow and caused the terrible flooding that caused the loss of life the Tbilisi landslide and flood disaster:.
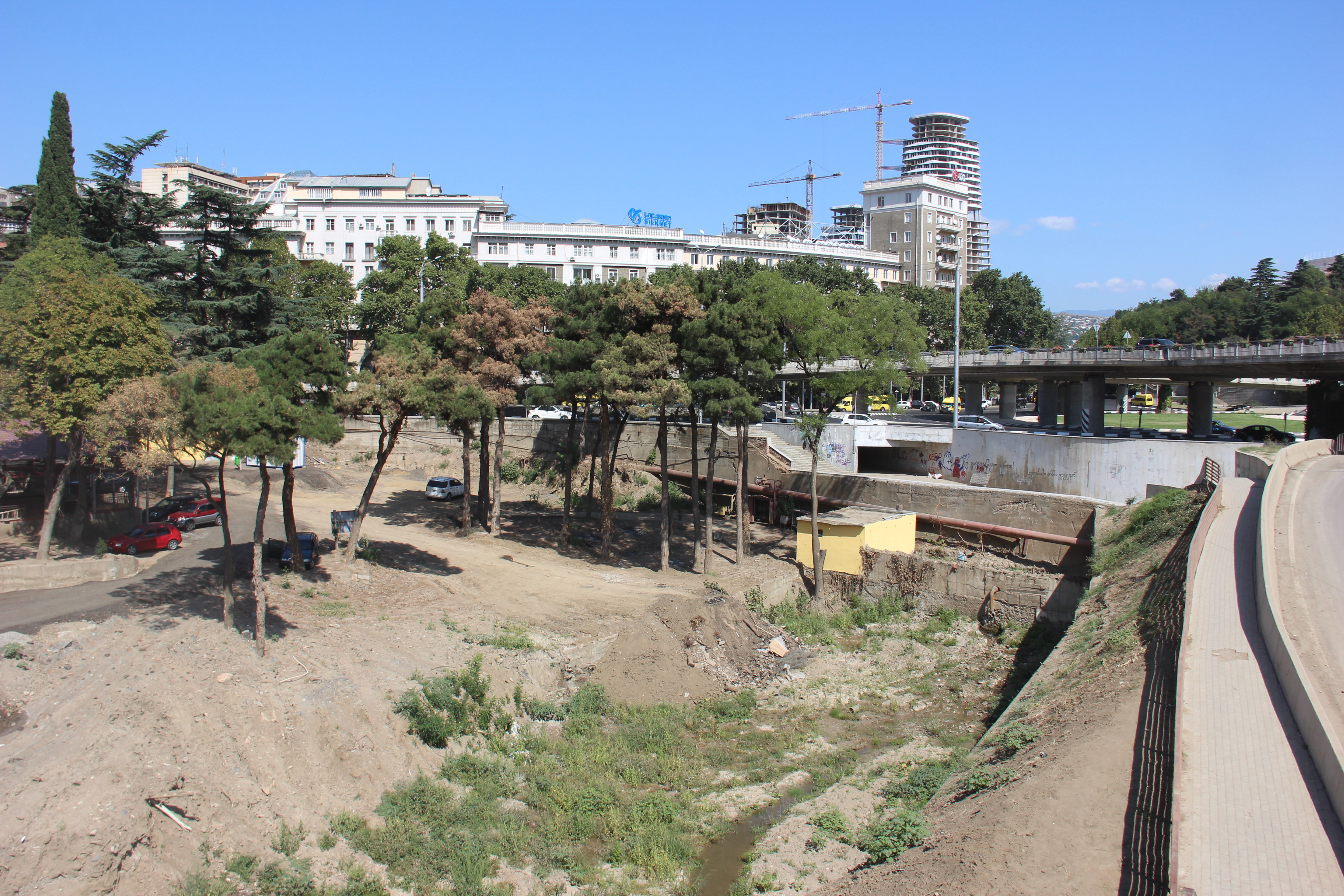
The new road junction at Gmirta Moedani (Heroes Square) that dammed the flow. Image courtesy of Dr Sergey Chernomorets
.
This is the site of the zoo that was so profoundly damaged:
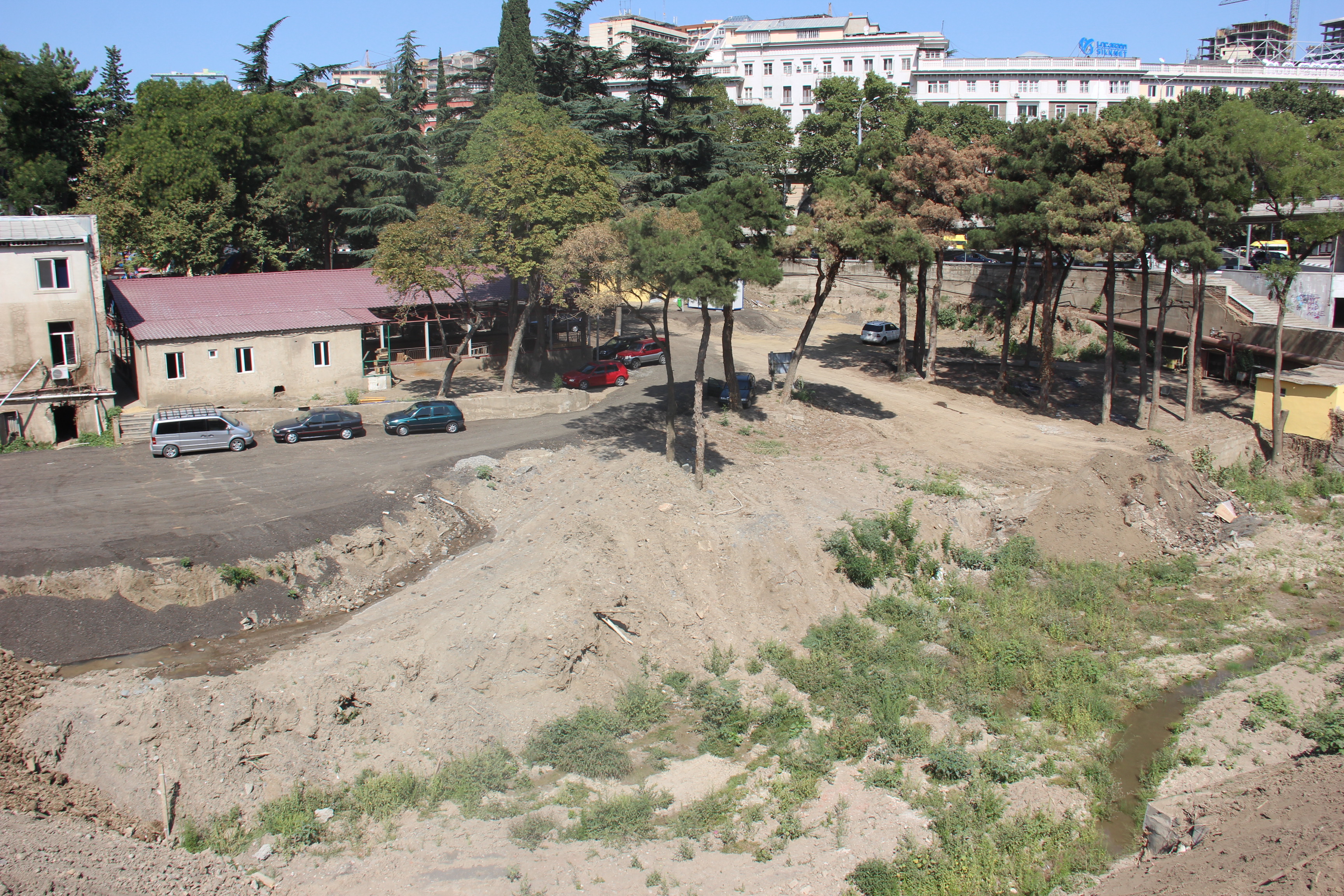
The site of Tbilisi Zoo courtesy of Dr Sergey Chernomorets
.
And this image is an example of the damage to the buildings in this area of Tbilisi. Note the height of the flood water and sediment on the walls:
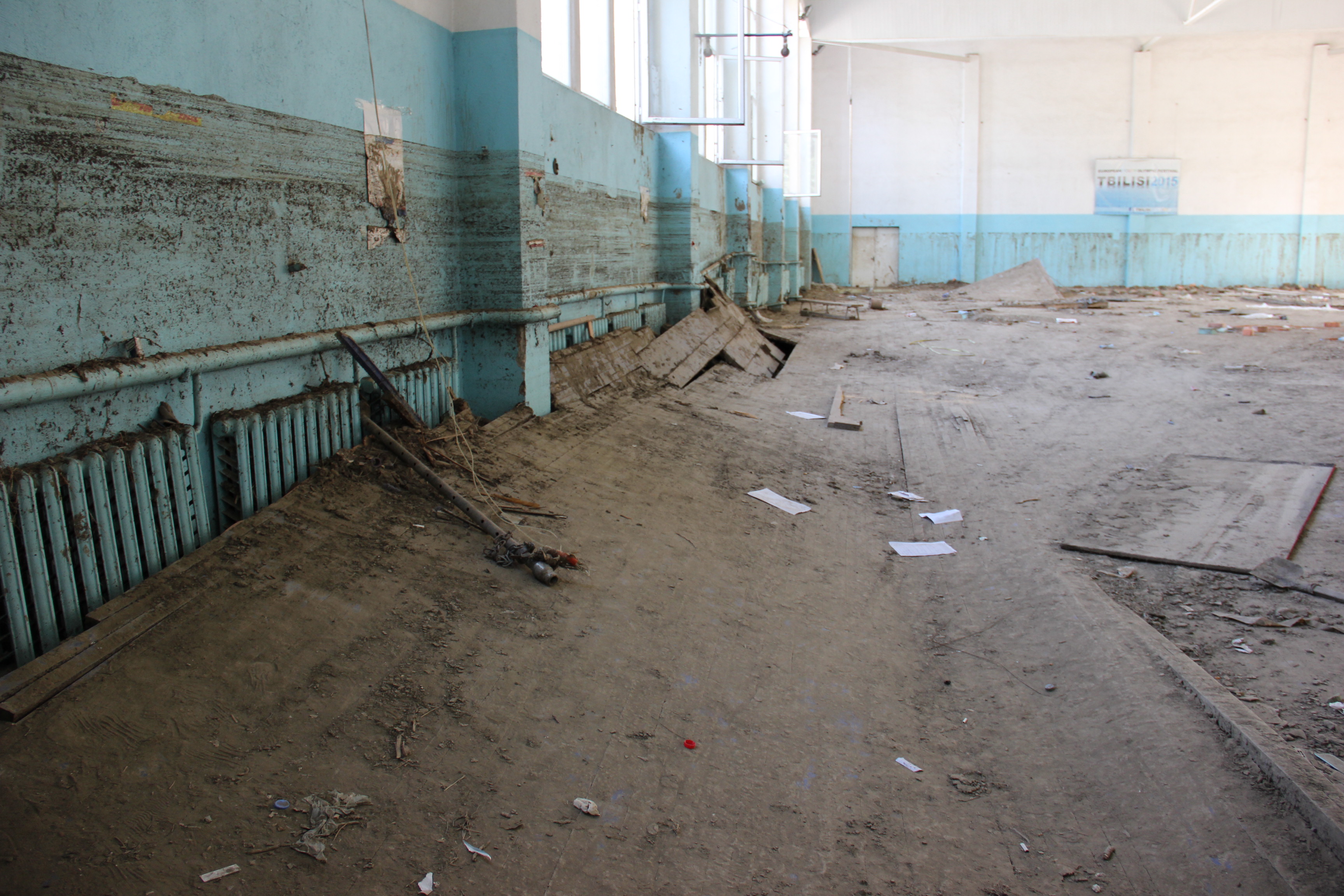
Building damage from the Tbilisi zoo landslide and flood disaster courtesy of Dr Sergey Chernomorets
28 January 2016
Google Earth imagery of the Tbilisi landslide and flood
Google Earth imagery of the Tbilisi landslide and flood
On 14th June 2015 a major flood swept through parts of Tbilisi in Georgia, causing the loss of 19 lives. The greatest damage associated with the Tbilisi landslide occurred at Tbilisi zoo – I blogged about this a few days later because the reported cause of the flood was a landslide that had blocked the valley, allowing a lake and then a dam break flood to develop. I noted at the time that there was a lack of clarity about exactly where the landslide that caused the disaster had occurred. Google Earth has now posted imagery of the area collected on 24th June 2015, about ten days after the disaster. This is an overview of the affected area from the south, the outskirts of Tbilisi are on the bottom left of the image:
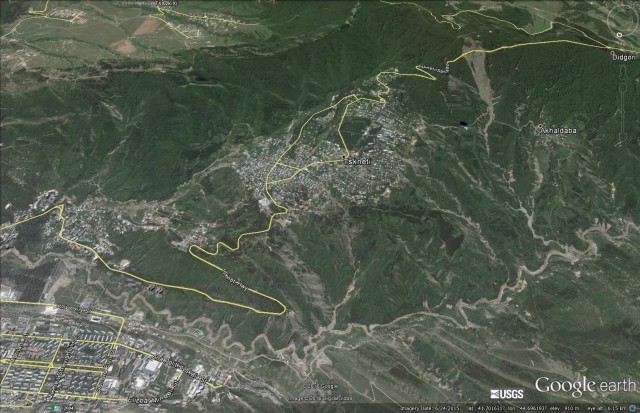
An overview of the Tbilisi landslide and flood disaster site via Google Earth
.
The most obvious feature here is a major landslide in the upper right portion of the image, with many smaller landslides in the vicinity. The path of the flood along the river is also clear. This is the same location in March 2014:
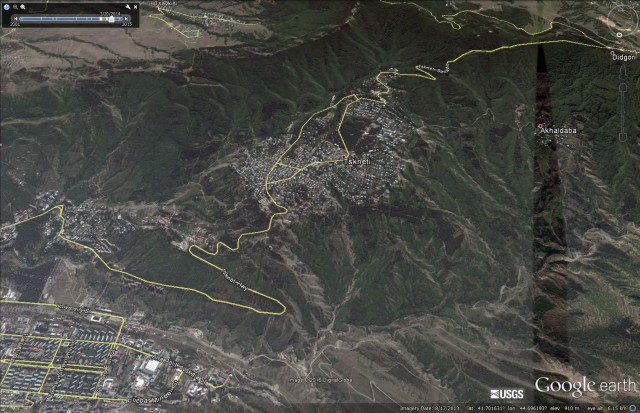
A Google earth image of the location of the Tbilisi landslide and flood from March 2014
.
The major feature is the large landslide that reportedly caused the disaster. This is a substantial feature that appears to be a comparatively shallow but extensive translational rock and soil slide. The main source appears to be in the vicinity of the road that crosses the upper part of the slope:
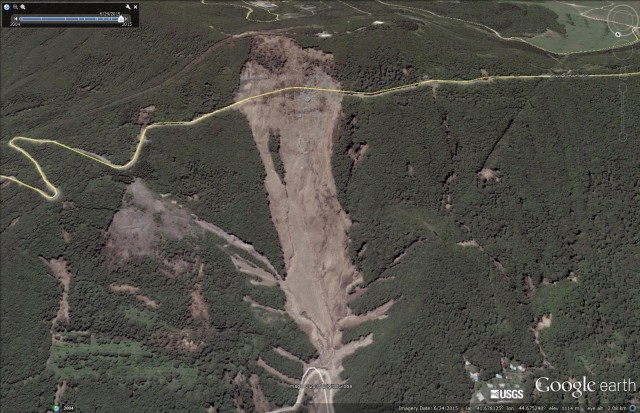
Google Earth image of the Tbilisi landslide
.
This landslide is about 1 km long and 300 metres wide at its maximum extend
The pre-landslide image of the same location gives little indication of the potential problems at this site, and there is no obvious sign that the road played a part in the failure:
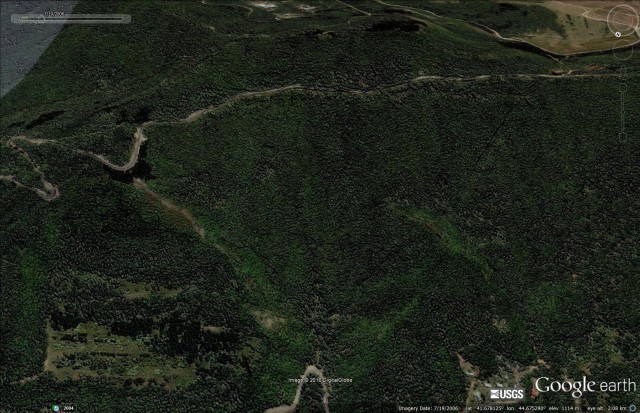
Google earth image of the site of the Tbilisi landslide
.
However, it is not obvious to me from the image that there was a major landslide dam event in this area, at least not associated with this landslide. Downstream there appears to be extensive evidence of shallow landslides and extensive flood scour on the river, but no obvious evidence of a dam and lake.:
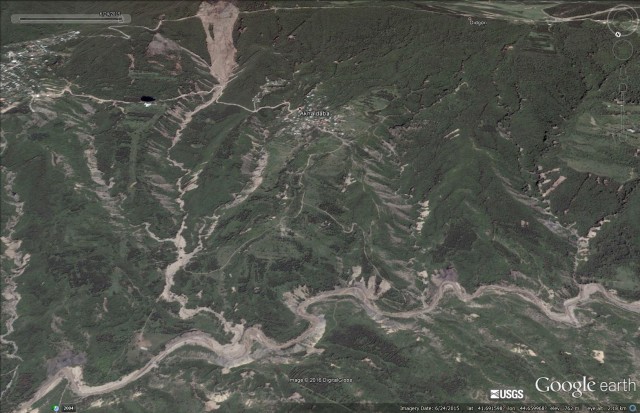
Google Earth imagery of the Tbilisi landslide site
.
Thus, to me on first inspection it appears that the disaster might have been caused by a large debris flow and flood that originated in the landslide shown above, with contributions from many other smaller landslides and channels, rather than a valley blocking event.
27 January 2016
Landslides in Chile 4: The Punta Cola rock avalanche in Aysén Fjord
The Punta Cola rock avalanche in Aysén Fjord, Chile
By Dr Melanie Froude (@Froudo_baggins), University of East Anglia
Aysén (Aisén) fjord is located at 45.26° latitude in Zona Austral, Chile (Chilean Patagonia). In common with other mountainous regions in Chile, the landscape is tectonically controlled, however in contrast to valleys such as the Maipo valley in the Central Andes, the coastline of south Chile is dominated by fjords. The fjords were formed during deglaciation, when rising sea levels inundated glacier-carved valleys as the continental ice cap retreated towards the end of the Pleistocene (about 15,000 years ago). Erosion by the ice has left deep fjords (Aysén fjord has a depth greater than 350 m), steep bedrock slopes and ice covered mountain peaks, with lower level hummocky terrain attributed to glacial moraines and landslides. Topography is overlain with shallow volcanic soils and temperate dense virgin forest, which thrives in the wet climate; Puerto Aysén has an average annual rainfall of 2,600 mm although this may reach 4,000 mm in the fjord.
Nestled within a steep drainage on the north side of Aysén fjord is the Punta Cola rock avalanche. Triggered by the Mw 6.2 Aysén earthquake on 21 April 2007 on the Liquiñe-Ofqui Fault zone (LOFZ), this was the largest of a total 538 mapped earthquake-triggered landslides ( Sepúlveda and Serey 2009; Sepúlveda et al., 2010), with an estimated total volume of 22.4 M m³ (Oppikofer et al., 2012).
Without a site visit, the magnitude of this landslide is difficult to comprehend in real terms; the panorama and insert below, illustrate quite how small ‘we’ are and present an intimidating reminder of the extent of damage caused when large landslides occur in densely populated regions.
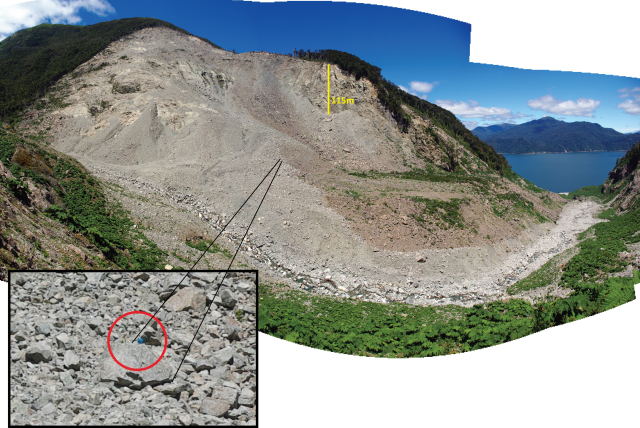
The main scar of the Punta Cola rock avalanche and the valley infill of rock avalanche deposit viewed from the north-west valley slopes, looking north east. Insert: shows a researcher sat on-top of a ~4m boulder for scale
The Punta Cola rock avalanche deposit is composed of a very large event and seven smaller secondary landslides. The main rockslide was composed of a <135 m thick block of North Patagonian Batholith, which broke into small fragments, sliding into the valley and running up the opposite slope by up to 180 m (Hermanns et al., 2014). The slide transformed into a highly mobile rock avalanche travelling down valley for 1.5 km into Aysén fjord, generating a displacement wave (akin to a tsunami). The rock avalanche accelerated as it descended into the fjord causing a large section of the pre-existing shoreline sediment ramp to collapse. Bathymetric data obtained in March 2013 (see Hermanns et al., 2014) show deformation across the full 3.5 km fjord width, interpreted as the impact of the avalanche as it slammed into sediments on the fjord floor, generating up to 10 m of vertical erosion and deformation structures, including concentric compressional ridges and large (< 70 m) sediment fragments.
Oppikofer et al. (2012) used terrestrial LiDAR to create a high resolution topographic model (DEM) of the landslide scar and deposit, from which an estimate of landslide volume was obtained, and geomorphological units were mapped. They hypothesised that failure was initiated at compartment A (see below), triggered by the 2007 earthquake, which led to the sequential failure of compartments B and C shortly after the main event. Material from compartments A and B was thought to have contributed to the rock avalanche, while compartment C was likely to have deposited at the base of the slope, possibly temporarily damming the river valley.
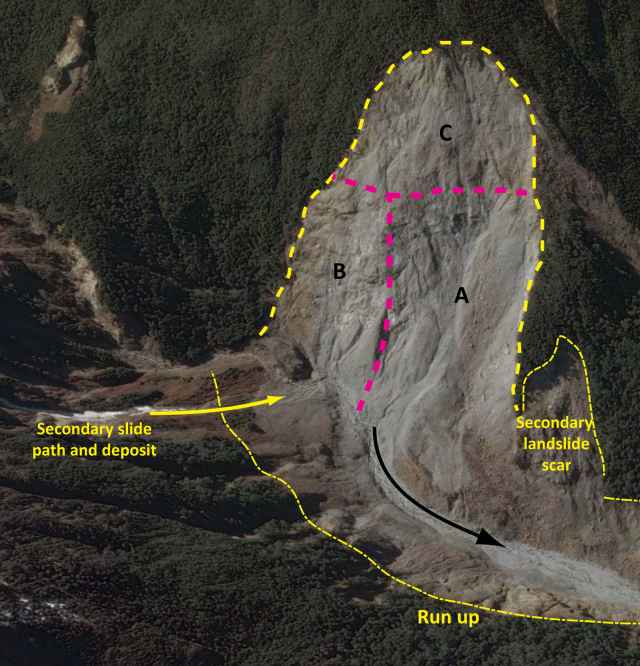
Compartments A, B and C within the Punta Cola rock avalanche scar. Redrawn from Oppikofer et al. (2012) interpretation onto a Google Earth basemap.
The evolution of the Punta Cola landslide scar and deposit are being monitored by a team from NGU, headed by Reginald Hermanns, in collaboration with the University of Chile. The team repeated the terrestrial LiDAR survey earlier this month, and will be comparing the new data with their previous survey (from 2010) to identify recent landslide activity and measure changes in the morphology of the debris fan.
Large sections of slope eroded by the 2007 rock avalanche run-out are now revegetated, primarily by Gunnera Tinctoria (Chilean rhubarb), which seems to be the main colonizing plant on disturbed areas around the fjord. Debris slides and rockfall persist in the valley, primarily from unvegetated sections of loose boulders near the valley floor and from within the main and secondary bedrock scars, which are composed of highly fractured rock. Comparison to photographs in the Oppikofer et al. (2012) paper show that over 10 m of rock avalanche deposit has been remobilised, highlighting the transport capacity of water-sediment flows over just five years, in sediment-charged mountainous environments.
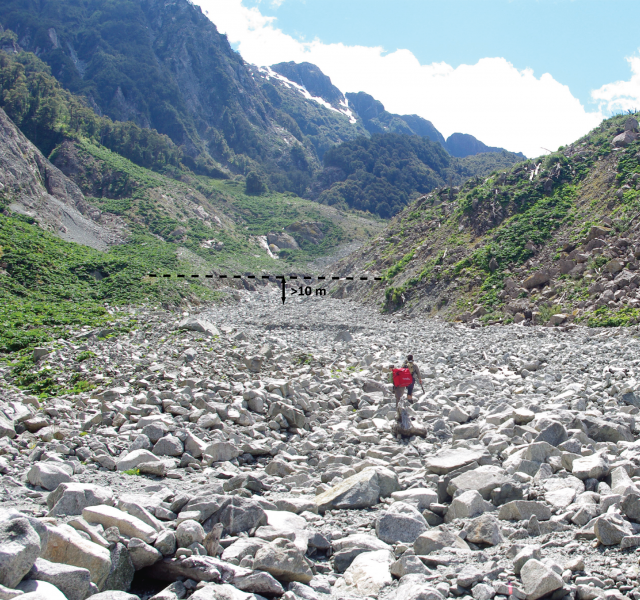
Looking upstream towards the base of the Punta Cola rock avalanche ~1 km from Aysén fjord. Over 10 m of material has been eroded from the 2007 rock avalanche and secondary landslide deposits by sediment-water flows between January 2010- January 2016.
The displacement wave from this landslide and a second wave from the Metirosa landslide (see Sepúlveda et al., 2010), caused significant damage to salmon farms in the fjord and to infrastructure on the shoreline (with run-ups of several tens of metres). Ten people were killed by these waves and debris flows associated with the earthquake. Operations in the fjord including fishing and tourism are of great economic value to the local community. Critically, the April 21 2007 Aysén earthquake is the first seismic event to be directly attributed to the LOFZ. Ongoing research is focused on the seismic and landslide hazard posed by future earthquakes on the LOFZ.
References
Hermmans, R.L., et al., (2014) Ch 14: Earthquake-triggered subaerial landslides that caused large scale fjord sediment deformation: combined subaerial and submarine studies of the 2007 Aysén fjord event, Chile. In: Lollino, G. et al. (eds.) Engineering Geology for Society and Territory – Volume 4. Doi: 10.1007/978-3-310-08660-6_14, Springer International Publishing, Switzerland
Oppikofer, T., Hermanns, R.L., Redfield, T., Sepúlveda, S.A., Duhart, P., Bascuñan, I. (2012) Morphologic description of the Punta Cola rock avalanche and associated minor rockslides caused by the 21 April 2007 Aysén earthquake (Patagonia, southern Chile). Revista Asociación Geológica Argentina 69:339–353.
Sepúlveda, S.A. and Serey, A. (2009) Tsunami-genic, earthquake-triggered rock slope failures during the April 21, 2007, Aisén earthquake, southern Chile (45.5° S). Andean Geology 36: 131-136. Doi: 10.4067/S0718-71062009000100010.
Sepúlveda, S.A., Serey, A., Lara, M., Pavez, A. and Rebolledo, S. (2010) Landslides induced by the April 2007 Aysén fjord earthquake, Chilean Patagonia. Landslides 7: 483-492. Doi: 10.1007/s10346-010-0203-2.
24 January 2016
The greatest ever debris flow video? Aconcagua in Argentina
The greatest ever debris flow video? Aconcagua
This one, on the flanks of Aconcagua in Argentina, is quite spectacular. It starts slowly, but hang in there!
.
.
The original text accompanying the video is in Spanish, a tidied up Google Translation version is as follows:
“Avalanche between Horcones (park entrance) and Confluence (first base camp of the Aconcagua field). Julian Insarralde [who posted the video], Nico Aguero and Naco Choulet were working for INOUT ADVENTURE. During a trek lasting three days. We are going to customers to avoid them being splashed with mud as it is an area of avalanches at that time of year. The warning was a sound similar to an airplane sound, which is why Julian Insarralde is looking back and is able to warn that an avalanche is coming. That’s why we ran and we did not abandon people so that we were in the safe zone. They are things that can happen when we work in real natural environments”.
This is the moment that the debris flow arrives:-
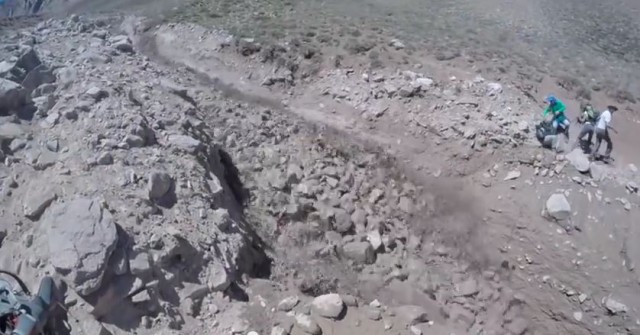
Aconcagua debris flow via Julian Insarralde and Youtube
.
This is a classic debris flow – the front end is almost entirely dry (note the dust in the image above) and mostly large boulders. The tail of the debris flow has more water and finer material. Note that the debris flow goes through a series of surges.
The weather appears to be dry and sunny. The very small debris flow that the hikers are crossing at the start is also quite intriguing. Even this appears to be debris rich. I wonder if this is the tail of an earlier surge? The walls of the gully appear to be wet?
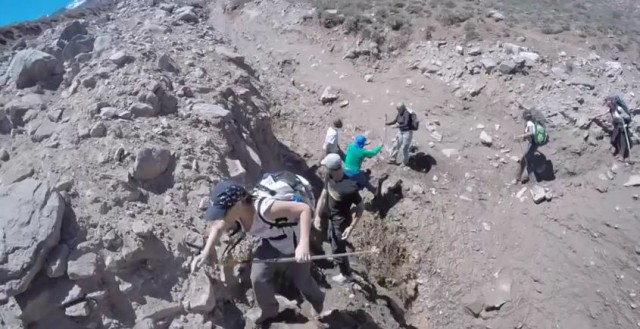
Aconcagua debris flow via Julian Julian Insarralde and Youtube
.
Its a pretty good job that the trekkers heard the debris flow coming, and respect to everyone for getting out of the way, despite a couple of slips and trips.
Recent Comments